We aim to understand macroscopic phenomena by elucidating the structure of materials on the Earth surface and their formation mechanisms. The following are some of our recent research topics.
Elucidation of biomineralization mechanisms
Organisms often utilize inorganic materials (biominerals) as their hard tissues like bone, teeth, exoskeleton, shell, scale, etc. It is well known that our bone and teeth are made of calcium phosphates (e.g., hydroxyapatite) with organic matrices. On the other hand, shell of mollusks, exoskeleton of crustacean, eggshell, and otolith are made of calcium carbonate. These hard tissues often exhibit excellent properties to sustain the activity of the organisms, which are generally originated from well regulated micro- (or nano-) structures (crystalline phase, size distribution, morphology, defect, crystal orientation) of the biominerals in the tissues. Another characteristic of the biominerals is that they are formed in environments where organisms live, i.e., at ambient temperature and pressure, and relatively in a short time, which is largely different from minerals in rocks. This means that kinetics often play an important role with thermodynamics. The formation and regulation mechanisms of biominerals have been investigated for a long time, but still not well understood.
It is known that most biominerals nucleate on organic matrices which may regulate the crystal orientation and possibly crystalline phase, and they grow in solutions incorporating organic molecules secreted from the organism. These intracrystalline organic molecules may also influence the structure of biominerals. We are investigating the roles of these organic matrices and intracrystalline molecules in the formation processes of biominerals, by analyzing fine structures in the hard tissues mainly using electron microscopy, and by conducting in vitro experiments of crystal growth. The research of biominerals is definitely an interdisciplinary science. We often collaborate with other laboratories with different methodologies to advance the research.
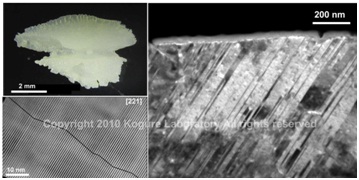
(Top left) Optical micrograph of otolith in salmon. (Right) Dark-field TEM image of the otolith to show high density of {110} aragonite twins. (Bottom-left) HRTEM image of the twins.
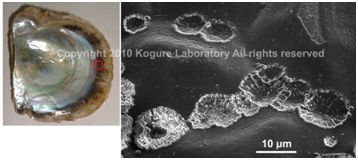
SEM image of the initial calcified structure in the nacreous layer of a pearl oyster,Pinctada fucata(left), formed on the organic sheet which covers the pre-existing prismatic outer layer. (Saruwatari et al., 2009)
Elucidation of contamination caused by the Fukushima nuclear accident
To solve the radioactive contamination caused by the Fukushima Dai-ichi Nuclear Power Plant accident in March 2011, an in-depth understanding of the Earth surface materials has been required. Our laboratory has obtained many results to clarify the actual situation of contamination due to radiocesium released from the damaged reactors. For example, we have identified specific minerals that selectively adsorb radiocesium deposited on soil by analyzing actual soil samples and conducting laboratory experiments. In particular, we showed that weathered biotite (partially vermiculitized biotite), which is abundant in the granitic soil that is common in Fukushima, efficiently adsorbs and fixes radiocesium. Furthermore, since the sorbed radiocesium is not readily released, decontamination by mild processes is difficult, whereas the transfer of radiocesium to groundwater and plants is significantly limited. Also, we have recently been investigating the structure and stability in the environment of radioactive glass microparticles that were directly emitted from the damaged reactors.
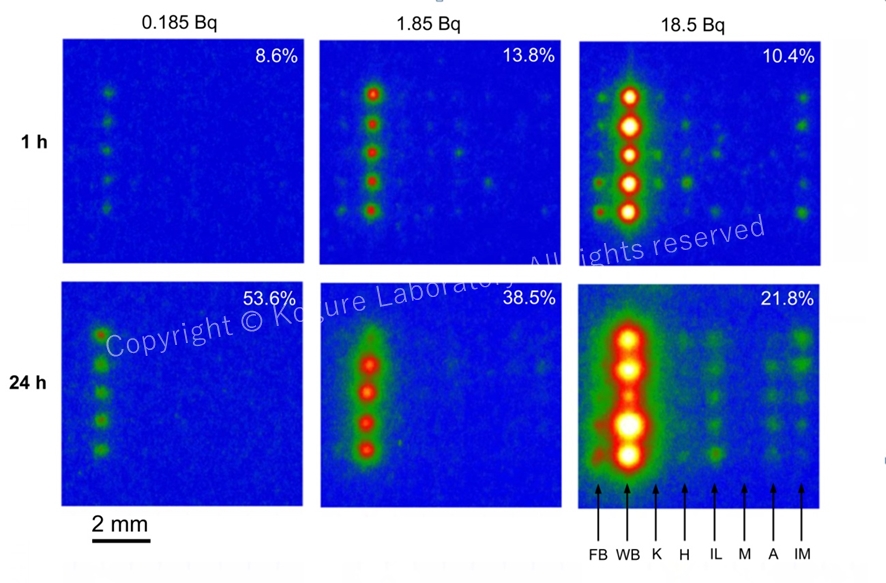
Imaging plate (IP) read-out images exposed to various mineral particles sorbing radiocesium (five particles of the same mineral are placed vertically). The radioactivity of137Cs is shown on the top and the reaction time on the left. FB: fresh biotite. WB: weathered biotite. K: kaolinite. H: halloysite. IL: illite. M: montmorillonite. A: allophan. IM: imogolite. This result shows that WB adsorbs Cs much more efficiently than other minerals. (Mukai et al., 2016)
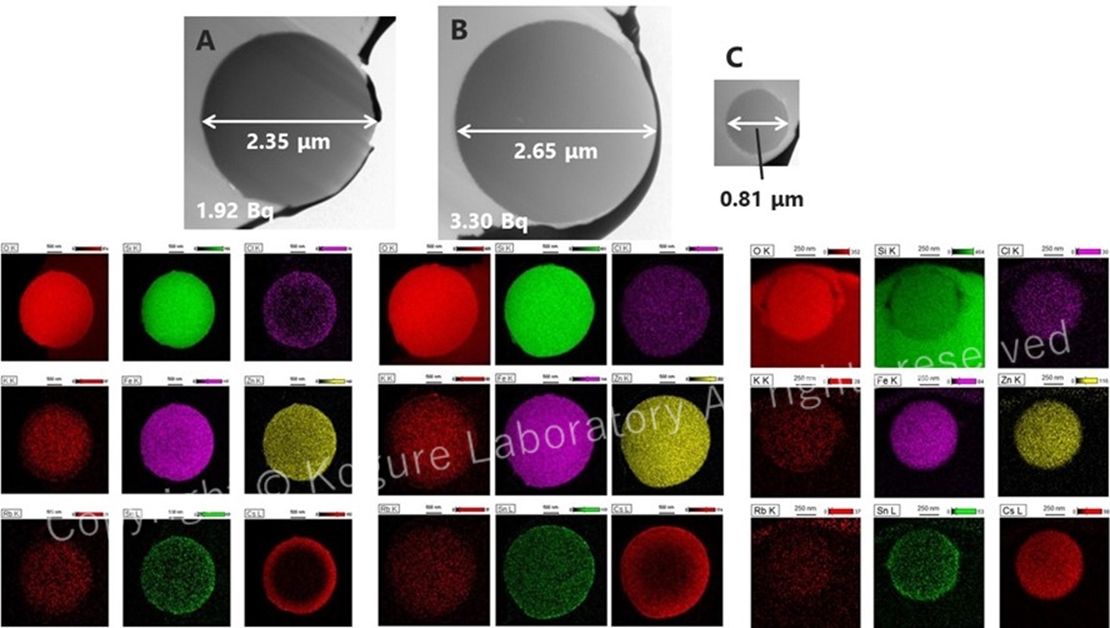
TEM images and elemental maps acquired using STEM of radioactive silicate glass microparticles (radiocesium-bearing microparticles, CsMPs) released directly from the damaged reactors. The Cs distribution in the lower right is inhomogeneous for some particles and is concentrated near the surface. (Kogure et al., 2016)
Fine structures of clay minerals and related layered materials
If the minerals formed at a high temperature and/or pressure deeply under the ground are exposed to surface environments, they decompose and transform to new minerals, which are often called “secondary minerals.” Clay minerals are representative secondary minerals abundant on the earth or in biosphere. Clay minerals form as very fine particles which we cannot recognize visually or even if using optical microscopy. However, they are very important and intimate materials for our life. For instance, clays are main inorganic constituents of soil, where they retain water, supply nutrients, or adsorb toxic elements. Clays are the raw materials of ceramic industries, and furthermore, can be new materials for future, sustainable society. Many clay minerals belong to phyllosilicates (or sheet silicates). The structures of phyllosilicates are characterized by infinite sheets made of SiO4 tetrahedrons. Moreover, the tetrahedral sheets combine with octahedral sheets, alkaline metals, water molecules, etc. to form a layer as a structure unit of phyllosilicates. The internal structure of the unit layer is rather rigid, but mutual position between the adjacent layers is often variable, these variables result in the formation of “polytypes”, a special case of polymorphs for layered materials, and/or dense stacking faults.
As mentioned above, clay minerals are so fine and their stacking structures can be varied that their true structures have not been well understood yet. Traditionally powder X-ray diffraction, thermal analyses, infrared spectroscopy, etc. are applied to identify or investigate the structures of clays. However, such “macroscopic” analyses often do not give solutions for the understanding of the clay structures. On the other hand, high-resolution transmission electron microscopy (HRTEM) is a powerful tool to determine the clay structures by observing directly their atomic structures, from which we can readily understand polytypes, stacking faults, interstratification in clays. However, HRTEM of clays has several obstacles. Radiation damage by electron beam is the most serious one. We are challenging to reveal the true structures of clays, by applying state-of-the-art electron microscopy to them and overcoming these obstacles.
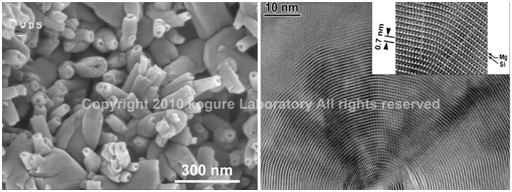
(Left) SEM image of halloysite tubes formed from weathering of feldspar. (Right) HRTEM image of the cross-section of a chrysotile tube (polygonal serpentine).
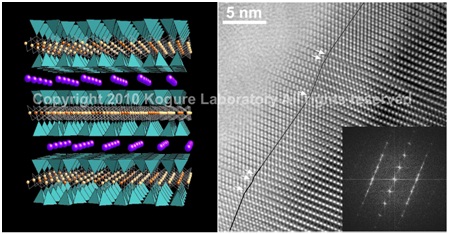
(Left) Crystal structure of trioctahedral mica. (Right) HRTEM image of kaolinite with enantiomeric twins domains.
Developments of new techniques using electron beam to investigate minerals
Most minerals are crystalline. Crystals are characterized with the repeated units in their atomic structures, termed “unit cell”. After the discovery of X-ray and subsequent establishment of diffraction theory, people devoted to determine the atomic arrangement in the unit cell in minerals, mainly using X-ray diffraction. Owing to their enormous efforts, the unit cell structures of most minerals have been precisely determined in the last century, which is the fundamental knowledge to understand the properties of minerals. However, the atomic arrangement in the unit cell represents only the “average” structure of the whole crystal and is not enough to explain various phenomena in mineralogy. For instance, morphology of minerals is primarily determined by the surface energy of crystals, and the surface energy depends on the atomic arrangement at the surfaces to terminate three-dimensional crystal structures. However, the surface atomic structures of minerals are generally not well understood yet. Including such surface structures, understanding of local structures in minerals is necessary for the advance of mineralogy and terrestrial material science. For this purpose, new ideas, methods, or techniques must be introduced or developed in this science field, which another subject of our laboratory.
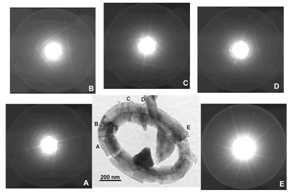
Application of orientation analyses using Kikuchi-patterns generated by a convergent beam, to biominerals. (Saruwatari et al., 2008)